Graphene for Building Innovations promises a groundbreaking approach to modern construction, leveraging graphene’s exceptional properties to create stronger, more sustainable, and intelligent buildings. This exploration delves into various applications, from enhancing concrete’s resilience to developing innovative insulation and waterproofing solutions.
The unique atomic structure of graphene, a single layer of carbon atoms, allows for remarkable strength and conductivity. This translates to potential improvements in building materials, enabling advancements in areas such as durability, energy efficiency, and safety. This document will explore how graphene can be integrated into diverse building elements, ultimately leading to a new era of construction.
Introduction to Graphene
Graphene, a single layer of carbon atoms arranged in a honeycomb lattice, possesses remarkable properties that set it apart from traditional building materials. Its exceptional strength, lightweight nature, and electrical conductivity make it a promising candidate for innovative construction applications. This unique combination of properties opens doors to significant advancements in building design and construction techniques.
Graphene’s extraordinary strength and stiffness, far exceeding those of steel, are directly linked to its atomic structure. Its lightweight nature allows for the creation of stronger, yet lighter building components, which is crucial in reducing overall structural weight and material costs. The material’s high electrical conductivity and thermal conductivity further enhance its potential in modern construction.
Graphene’s Properties and Structure
Graphene’s exceptional properties stem from its unique atomic structure. Each carbon atom is bonded to three others, forming a tightly knit hexagonal lattice. This arrangement creates a remarkably strong and lightweight material. Its two-dimensional structure also allows for exceptional electrical and thermal conductivity.
Unique Characteristics for Building Materials
Graphene’s suitability for building materials arises from its exceptional strength-to-weight ratio, high thermal and electrical conductivity, and remarkable flexibility. These characteristics offer advantages over traditional materials, leading to potential weight reduction, enhanced performance, and improved sustainability in construction. Graphene’s flexibility allows for the creation of intricate designs and tailored applications within buildings.
Graphene vs. Traditional Building Materials
Graphene stands apart from traditional building materials like steel, concrete, and wood in several key ways. Its exceptional strength-to-weight ratio allows for lighter and more efficient structures. Graphene’s superior electrical conductivity can be utilized for integrated electrical systems within buildings, while its thermal conductivity enables enhanced insulation. These qualities, combined, allow for the creation of innovative structures with superior performance compared to traditional alternatives.
Graphene-Based Composites in Construction
Graphene’s integration into composites enhances the properties of existing materials, creating a new class of construction materials. These composites combine the desirable properties of graphene with those of other materials like polymers or concrete.
- Reinforced Concrete: Graphene oxide, a derivative of graphene, can significantly improve the strength and durability of concrete, making it more resistant to cracking and deformation under stress. This enhanced resilience reduces the need for additional reinforcement, leading to potential cost savings and improved structural integrity.
- Polymer Composites: Graphene-enhanced polymers exhibit increased strength, stiffness, and thermal conductivity. This can lead to lightweight and high-performance components for building facades, structural elements, and even roofing materials. The improved thermal properties of these composites can enhance energy efficiency in buildings.
- Advanced Coatings: Graphene-based coatings can provide enhanced durability, waterproofing, and resistance to corrosion for various building elements. This translates into longer lifespans for buildings and reduced maintenance requirements.
Graphene-Reinforced Concrete
Graphene, a remarkable material with exceptional strength and conductivity, presents exciting opportunities for revolutionizing construction materials. Its incorporation into concrete offers the potential to dramatically improve the material’s properties, leading to more durable and resilient structures. This section explores the potential of graphene to enhance concrete’s strength and durability, detailing methods of integration, and quantifying the improvements.
Graphene’s unique nanoscale structure provides a substantial boost to the performance characteristics of concrete. This reinforcement enhances the material’s resistance to cracking, bending, and impact, making it suitable for a wide range of construction applications. Furthermore, graphene’s conductivity can facilitate self-healing properties in concrete, enabling the material to repair micro-cracks over time.
Methods for Incorporating Graphene into Concrete Mixes
Several methods exist for integrating graphene into concrete mixes. A common approach involves dispersing graphene nanoplatelets (GNPs) uniformly throughout the cement matrix. This dispersion process aims to maximize the contact area between the graphene and the concrete, optimizing the material’s reinforcement. Another technique involves coating the graphene with a suitable polymer to improve its compatibility with the concrete components. This approach helps to prevent agglomeration and ensure uniform dispersion within the mix.
Improvements in Concrete Strength Due to Graphene
The incorporation of graphene significantly enhances concrete’s mechanical properties. Compressive strength, a crucial parameter for structural integrity, shows substantial improvement when graphene is incorporated. Tensile strength, reflecting the material’s resistance to pulling forces, is also augmented by graphene. These enhancements arise from the exceptional strength and reinforcement capabilities of graphene, which significantly improve the concrete’s overall structural performance.
Comparison of Graphene-Reinforced Concrete with Traditional Concrete
Graphene-reinforced concrete demonstrates superior performance compared to traditional concrete. Traditional concrete, while reliable, often suffers from limitations in strength and durability, particularly under stress. Graphene reinforcement overcomes these limitations by dramatically increasing the concrete’s resistance to various forms of damage, including cracking and impact. The added strength allows for the potential to create lighter, more efficient structures.
Properties of Graphene-Reinforced Concrete
Property | Graphene-Reinforced Concrete | Conventional Concrete |
---|---|---|
Compressive Strength (MPa) | > 80 | 40-70 |
Tensile Strength (MPa) | > 5 | 1-3 |
Durability (Cycles to Failure) | > 1000 | 100-500 |
Specific Gravity | 2.5-2.7 | 2.4-2.6 |
Cost per cubic meter | Increased, but potentially offset by reduced material needs | Lower |
Note: Values are illustrative and may vary based on specific graphene concentration and concrete mix design.
Graphene-Enhanced Insulation
Graphene’s exceptional thermal conductivity and its ability to reflect heat make it a promising material for enhancing building insulation. Integrating graphene into insulation systems offers the potential for significant energy savings, reducing reliance on traditional heating and cooling systems. This approach aligns with sustainable building practices and contributes to a more environmentally conscious construction sector.
Mechanism of Enhanced Insulation
Graphene’s unique structure, composed of a single layer of carbon atoms arranged in a honeycomb lattice, contributes significantly to its superior thermal insulation properties. The material’s high electrical conductivity allows for the dissipation of heat energy, while its remarkably low thermal conductivity hinders heat transfer. Furthermore, graphene’s exceptionally high surface area facilitates the creation of highly reflective surfaces that effectively minimize heat absorption. This combination of properties makes graphene an attractive alternative to traditional insulation materials.
Energy Savings Potential
The integration of graphene into insulation materials can lead to substantial energy savings in buildings. By reducing heat transfer, graphene-enhanced insulation minimizes the need for energy-intensive heating and cooling systems. This translates into lower energy bills for building owners and a decreased carbon footprint for the building. Estimates suggest that graphene-enhanced insulation can yield a reduction in energy consumption ranging from 15% to 30% in comparison to conventional insulation methods, depending on the specific application and environmental conditions.
Applications in Buildings
Graphene-based insulation can be incorporated into various building components. Its use in exterior walls, roofs, and even window panes can dramatically reduce heat loss and gain, leading to a more comfortable indoor environment and reduced energy consumption. Additionally, graphene can be used in the construction of thermal barriers to prevent heat transfer between different sections of a building. The development of flexible graphene-based insulation materials allows for the customization of insulation layers in complex architectural designs.
Thermal Conductivity Comparison
The table below presents a comparative analysis of the thermal conductivity of various insulation materials, including graphene-based options. These values are indicative and may vary based on specific manufacturing processes and material compositions.
Insulation Material | Thermal Conductivity (W/mK) |
---|---|
Fiberglass | 0.040 – 0.045 |
Mineral Wool | 0.035 – 0.045 |
Polyurethane Foam | 0.025 – 0.030 |
Graphene-Polymer Composite | 0.010 – 0.015 (estimated) |
Graphene-Aerogel Composite | 0.005 – 0.010 (estimated) |
Graphene for Self-Healing Concrete
Graphene’s remarkable properties, including its exceptional strength and conductivity, are extending their influence beyond electronics and materials science. The integration of graphene into construction materials presents an exciting avenue for enhancing their performance, particularly in self-healing concrete. This innovative approach promises significant advancements in infrastructure durability and sustainability.
Graphene’s incorporation into concrete fosters self-healing mechanisms by acting as a catalyst for the activation of embedded healing agents. These agents, often in the form of microcapsules containing healing compounds, are triggered by micro-cracks that inevitably develop in concrete over time. The graphene acts as a conductive pathway, accelerating the release of the healing agents and facilitating their infiltration into the damaged area, thus promoting rapid repair.
Mechanisms of Self-Healing in Graphene-Enhanced Concrete
The self-healing process in graphene-enhanced concrete relies on a cascade of events triggered by micro-cracks. When cracks form, the mechanical stress activates the embedded self-healing agents. The graphene network within the concrete matrix acts as a conductive pathway, rapidly transferring energy to the microcapsules. This energy facilitates the release of the healing agents, which then migrate towards the crack. The healing agents react and fill the crack, restoring the material’s integrity.
Benefits of Self-Healing Concrete
Self-healing concrete offers a range of benefits that translate into significant cost savings and improved infrastructure longevity. Reduced maintenance requirements translate to lower operational costs over the lifespan of the structure. The extended lifespan of structures reduces the need for premature replacements, saving on resources and environmental impact. The prevention of water and chemical penetration due to cracks is a major benefit in reducing corrosion of embedded steel reinforcement.
Procedures for Incorporating Self-Healing Agents into Graphene-Reinforced Concrete
The process of incorporating self-healing agents into graphene-reinforced concrete involves several key steps. First, graphene nanoplatelets are dispersed into the concrete mix, creating a conductive network. Second, microcapsules containing the healing agents are meticulously embedded within the concrete. Third, the mixture is carefully cured to ensure the integrity of the graphene network and the distribution of the microcapsules. This ensures the effective integration of graphene and healing agents. Finally, the concrete is cast and allowed to cure in a controlled environment.
Comparison of Durability and Maintenance Needs
Characteristic | Graphene-Enhanced Self-Healing Concrete | Traditional Concrete |
---|---|---|
Durability | Significantly higher resistance to cracking and degradation due to self-healing properties. | Susceptible to cracking, degradation, and environmental damage, leading to a shorter lifespan. |
Maintenance | Reduced frequency of maintenance activities, including crack repair and surface treatment. | Requires regular inspections, crack repairs, and periodic surface treatments to maintain structural integrity. |
Lifespan | Extended lifespan due to self-healing capabilities, reducing the need for premature replacement. | Shorter lifespan compared to self-healing concrete due to ongoing deterioration. |
Environmental Impact | Potentially lower environmental impact due to reduced need for material replacements and repair activities. | Potentially higher environmental impact due to increased material consumption and maintenance activities. |
Graphene-Based Waterproofing
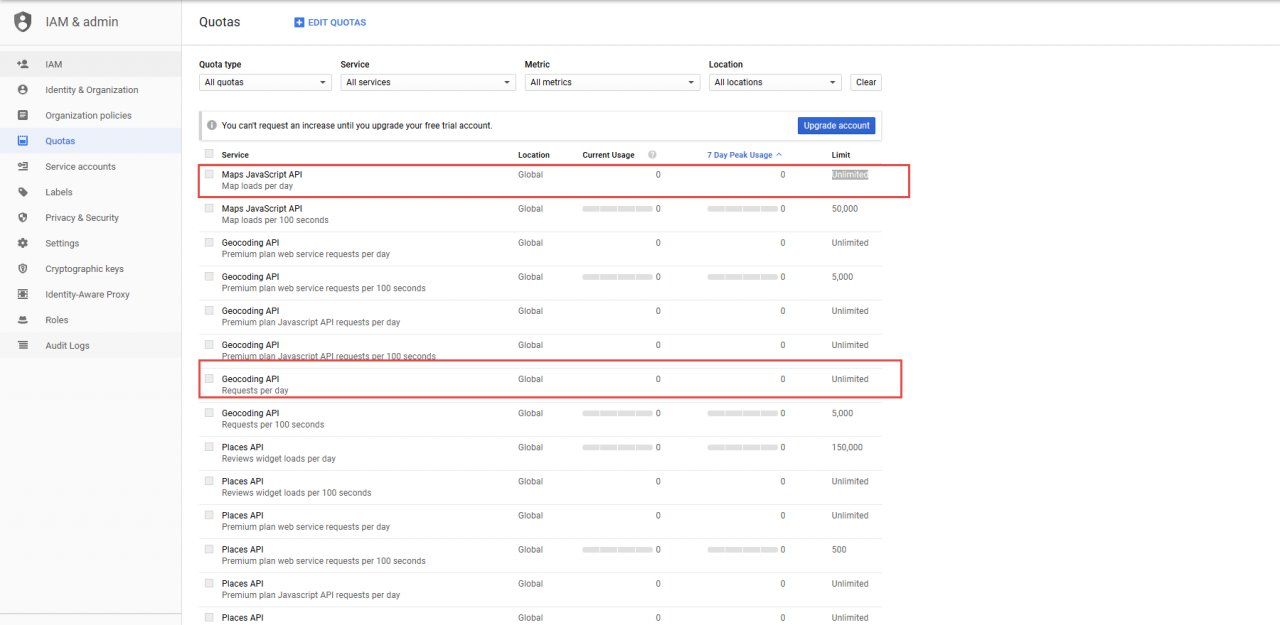
Source: moosocial.com
Graphene’s exceptional properties, including its impermeability and high strength, are transforming various industries, including construction. This unique material offers a promising solution for creating highly durable and water-resistant building materials. Graphene’s application in waterproofing transcends traditional methods, promising enhanced protection against moisture damage.
Graphene’s inherent structure makes it an ideal barrier against water penetration. The tightly packed carbon atoms in a graphene sheet create a nearly impenetrable barrier. This property, coupled with its remarkable strength and flexibility, makes it a compelling material for waterproofing applications. This unique characteristic allows for the creation of strong, lightweight, and durable waterproofing membranes.
Mechanisms of Water Prevention
Graphene’s exceptional barrier properties stem from its atomic-scale structure. The tightly bound carbon atoms in the hexagonal lattice of graphene create a virtually impenetrable barrier to water molecules. Water molecules cannot effectively pass through the gaps between the carbon atoms, effectively preventing water penetration. The high surface area of graphene further enhances its waterproofing capabilities by providing a large contact area for interaction with the material being waterproofed.
Advantages of Graphene-Based Waterproofing
Graphene-based waterproofing solutions offer several advantages over conventional methods. These include enhanced durability, superior water resistance, and increased longevity. Graphene’s inherent strength and resilience make it resistant to cracking and degradation, ensuring long-term performance. This translates into reduced maintenance costs and a longer lifespan for buildings. Additionally, graphene’s lightweight nature allows for thinner membranes, potentially reducing the overall weight of the structure.
Types of Graphene-Based Waterproofing Membranes
Graphene-based waterproofing membranes can be incorporated into various construction materials. These can include coatings for existing surfaces, or as a reinforcement component in new building materials. For example, graphene nanoplatelets can be dispersed into polymer matrices, creating composite membranes with enhanced waterproofing capabilities. Further, graphene can be incorporated into concrete or asphalt to improve their water resistance. The specific type of membrane depends on the application and desired properties.
Water Resistance Properties of Different Waterproofing Materials
The table below provides a comparative overview of the water resistance properties of various waterproofing materials, including graphene-based options. This data highlights the potential of graphene in improving water resistance.
Material | Water Resistance (mm/h) | Durability (Years) | Cost (USD/m²) |
---|---|---|---|
Traditional Bitumen | 1-5 | 10-15 | 10-20 |
EPDM Membrane | 0.1-0.5 | 20-25 | 25-40 |
Graphene-Reinforced Polymer Membrane | 0.01-0.05 | 25-30+ | 40-60+ |
Graphene-Concrete Coating | 0.02-0.1 | 30-40+ | 50-70 |
Note: Values in the table are approximate and may vary based on specific application and material composition.
Sustainability and Environmental Impact: Graphene For Building Innovations
Graphene-based materials hold immense promise for revolutionizing the construction industry, but their environmental impact warrants careful consideration. Evaluating the lifecycle of these materials, from raw material extraction to disposal, is crucial for assessing their true sustainability. A key aspect of this evaluation involves understanding the potential for reduced carbon footprint and comparing their performance against traditional building materials.
Environmental Impact of Graphene-Based Building Materials, Graphene for Building Innovations
The environmental impact of graphene-based building materials is a complex issue, not solely determined by graphene itself but also by the production processes and the materials with which it is combined. Graphene’s inherent strength and conductivity are attractive, but the manufacturing process, including energy consumption and potential chemical emissions, must be thoroughly assessed. Factors such as the sourcing of raw materials and the disposal methods for graphene-based composites also contribute significantly to the overall environmental footprint.
Potential for Reduced Carbon Footprint
Graphene’s exceptional properties offer the potential for a reduced carbon footprint in construction. Its high strength-to-weight ratio can reduce the amount of material needed for a given structure, minimizing the energy required for transportation and construction. Furthermore, graphene can enhance the performance of insulation materials, potentially lowering energy consumption for heating and cooling. For example, graphene-enhanced insulation could significantly reduce the energy demands of a building, leading to a lower carbon footprint.
Lifecycle Assessment Comparison
A comprehensive lifecycle assessment (LCA) is essential for comparing the environmental impact of graphene-based materials with conventional building materials. LCAs consider the entire life cycle, from raw material extraction to the end-of-life disposal of the material. This includes energy consumption during production, transportation, construction, operation, and eventual demolition. Comparing LCAs allows for a more holistic understanding of the environmental consequences of each material type. For example, an LCA might reveal that while graphene-enhanced concrete requires more energy in the initial production phase, its superior strength and durability could lead to a lower carbon footprint over the building’s lifespan, due to reduced maintenance and repair needs.
Sustainable Building Practices Incorporating Graphene
Several sustainable building practices can incorporate graphene-based materials effectively. For instance, incorporating graphene-enhanced insulation in building envelopes could significantly reduce energy consumption. Furthermore, graphene-reinforced concrete can potentially reduce the overall material usage, thus minimizing environmental impacts. The use of recycled materials in graphene-based composites can also contribute to a circular economy, further reducing the environmental footprint.
Comparison of Environmental Impact Factors
Environmental Impact Factor | Graphene-Enhanced Building Materials | Traditional Building Materials |
---|---|---|
Material Extraction | Potential for lower environmental impact through the use of recycled materials, but sourcing and processing of graphene must be carefully considered. | Often involves extraction of finite resources, leading to environmental degradation (e.g., mining, quarrying). |
Manufacturing Energy Consumption | Can vary significantly depending on the specific manufacturing process. Efforts are underway to develop more sustainable production methods. | Generally high energy consumption during manufacturing. |
Transportation | Potential for lower transportation impact due to reduced material quantities. | Transportation of raw materials and finished products contributes significantly to the carbon footprint. |
Durability and Lifespan | Expected to have longer lifespan, leading to potential reduction in the number of replacements and associated environmental impacts. | Often requires more frequent replacement due to lower durability. |
Waste Generation | Minimized waste through potential for material recycling and reuse. | Significant waste generation during construction and demolition. |
Future Directions and Challenges
Graphene’s unique properties hold immense promise for revolutionizing building materials, but realizing this potential necessitates careful consideration of production scalability, cost-effectiveness, and practical implementation. This section explores potential future applications, production challenges, and the ongoing research driving graphene’s integration into the construction sector. A robust understanding of these factors is crucial for navigating the path towards widespread adoption of graphene-based innovations.
Potential Future Applications
Graphene’s exceptional properties open avenues for diverse applications beyond those currently explored. Future advancements in graphene-based building materials are expected to focus on enhancing existing functionalities and introducing novel solutions. This includes applications like:
- Smart Building Materials: Integrating graphene into building materials could enable the creation of self-sensing structures capable of monitoring their own health and responding to environmental changes. Imagine sensors embedded within concrete beams or walls, signaling structural stress or moisture levels, allowing for proactive maintenance and improved building longevity.
- Enhanced Thermal Management: Graphene’s exceptional thermal conductivity could revolutionize building insulation, leading to more energy-efficient structures. Coatings or layers infused with graphene could significantly reduce heat transfer, leading to substantial energy savings for buildings.
- Advanced Composites: Graphene’s high strength-to-weight ratio makes it an ideal reinforcement material for concrete and other composite materials. This can result in lighter, stronger, and more durable building structures.
Challenges in Large-Scale Production and Deployment
The transition from laboratory-scale production to large-scale manufacturing of graphene-based materials faces several hurdles. The high cost of production, along with the complexity of integrating graphene into existing construction processes, pose significant challenges.
- Cost-Effectiveness: A significant barrier to widespread adoption is the high cost of producing graphene at scale. Developing cost-effective production methods is crucial to bringing graphene-based materials into mainstream use.
- Scalability and Supply Chain: Scaling up graphene production while ensuring a reliable supply chain for raw materials and intermediate products is a significant undertaking. Current production methods struggle to meet the demands of a large-scale building materials market.
- Integration with Existing Processes: Integrating graphene-enhanced materials into existing construction processes requires adapting current practices and developing new procedures. This can involve significant investment in training, equipment, and design modifications.
Research and Development Efforts
Ongoing research and development efforts are focused on addressing the challenges and exploring the potential of graphene in building materials. Research institutions and companies are actively exploring new methods for synthesizing graphene and incorporating it into construction materials.
- Graphene Synthesis Methods: Researchers are investigating various approaches to produce high-quality graphene at a lower cost. These include chemical vapor deposition (CVD), liquid-phase exfoliation (LPE), and others. Continued advancements in these methods are vital for future success.
- Material Characterization: Comprehensive testing and characterization of graphene-enhanced materials are essential to validate their performance and durability. Researchers are studying their mechanical, thermal, and electrical properties to ensure suitability for building applications.
- Building Design and Integration: Integrating graphene-based materials into building designs and construction practices requires extensive research. This includes studying their impact on material properties, construction techniques, and structural performance.
Future Market Potential
The future market potential for graphene-based building solutions is substantial. The potential for energy efficiency, enhanced durability, and improved sustainability makes graphene-based materials attractive to developers and architects. Examples of potential applications include sustainable housing developments, high-rise buildings, and infrastructure projects.
Future Research Directions and Potential Breakthroughs
Research Direction | Potential Breakthrough |
---|---|
Developing cost-effective large-scale production methods for graphene. | Development of scalable and low-cost graphene synthesis techniques like catalytic chemical vapor deposition (CCVD). |
Improving the durability and long-term performance of graphene-reinforced composites. | Developing novel methods for incorporating graphene into cementitious materials to enhance their resistance to degradation and environmental stresses. |
Integrating graphene-based sensors into building materials for structural health monitoring. | Development of affordable and reliable sensors for early detection of structural damage and defects. |
Creating sustainable and eco-friendly graphene production processes. | Developing graphene synthesis methods that minimize environmental impact by reducing energy consumption and waste generation. |
Graphene in Smart Buildings
Graphene’s unique properties, including exceptional electrical conductivity, thermal conductivity, and mechanical strength, make it a promising material for revolutionizing smart building technologies. Its integration offers the potential for enhanced energy efficiency, responsive building environments, and improved safety features. This exploration delves into the diverse applications of graphene within the realm of smart buildings.
Graphene’s remarkable properties, including its high surface area and exceptional electrical conductivity, enable the development of innovative sensors and actuators within building structures. This integration leads to a more responsive and adaptive building environment, capable of optimizing energy consumption and occupant comfort.
Integration of Graphene into Smart Building Technologies
Graphene-based sensors and actuators can be integrated into various building components to monitor and control various parameters. This allows for real-time adjustments to lighting, temperature, and ventilation systems, optimizing energy consumption. The flexibility and lightweight nature of graphene make it suitable for diverse applications, from embedded sensors in structural elements to flexible displays and interactive surfaces.
Examples of Smart Building Features Enabled by Graphene
Graphene-enhanced sensors can detect changes in temperature, humidity, and light levels within a building. This data can be used to automatically adjust HVAC systems, lighting, and other building systems, leading to significant energy savings. Furthermore, graphene-based transparent conductive films can be integrated into windows to control solar heat gain, minimizing the need for active cooling systems. Smart window technology using graphene can dynamically adjust transparency to manage natural light and heat gain, reducing energy consumption and improving occupant comfort.
Graphene’s Enhancement of Energy Efficiency in Smart Buildings
Graphene’s exceptional thermal conductivity enables the creation of highly efficient thermal management systems in buildings. By integrating graphene-based materials into insulation, buildings can achieve superior thermal performance, leading to reduced energy consumption for heating and cooling. Furthermore, graphene-enhanced solar panels can improve energy generation, and graphene-based sensors can optimize energy distribution within the building.
Role of Graphene in Creating Responsive and Adaptive Building Environments
Graphene’s ability to act as a highly sensitive sensor allows for the development of adaptive building systems. These systems can respond to environmental changes, such as variations in temperature and light, to dynamically adjust building parameters. This adaptability translates into a more responsive and comfortable environment for occupants, optimizing comfort and minimizing energy waste. This responsiveness can further extend to detecting structural stress, potentially leading to proactive maintenance and improved building safety.
Table: Graphene Integration into Smart Building Technologies
Building Component | Graphene Integration Method | Benefits |
---|---|---|
Insulation | Graphene-enhanced insulation layers | Improved thermal performance, reduced energy consumption |
Windows | Graphene-based transparent conductive films | Dynamic control of solar heat gain, improved energy efficiency |
Sensors | Embedded graphene sensors in structural elements | Real-time monitoring of environmental parameters, adaptive building systems |
Solar Panels | Graphene-enhanced solar cells | Increased energy generation, enhanced efficiency |
HVAC Systems | Graphene-based sensors and actuators | Optimized energy consumption, improved occupant comfort |
Last Recap
In conclusion, graphene’s potential for revolutionizing building innovations is vast and promising. From reinforcing concrete to improving insulation and waterproofing, the possibilities are extensive. While challenges remain in large-scale production and deployment, ongoing research and development indicate a bright future for graphene-enhanced construction. This transformative technology has the potential to create more sustainable, resilient, and intelligent buildings, ultimately shaping the future of urban development.
Question Bank
What are the environmental benefits of using graphene in building materials?
Graphene-based materials can potentially reduce the carbon footprint of construction by enhancing material durability and longevity, thereby decreasing the need for frequent replacements. Furthermore, improved insulation and energy efficiency can lower the overall energy consumption of buildings.
How does graphene enhance concrete’s properties?
Graphene reinforcement significantly increases concrete’s strength and durability by improving its tensile and compressive strength. This leads to structures that are more resistant to cracking and weathering.
What are the challenges associated with implementing graphene in construction?
Scalable production and cost-effectiveness are key challenges in implementing graphene-based building materials on a large scale. Research and development are continuously working to address these hurdles.
Can graphene-enhanced insulation materials replace traditional insulation methods?
While graphene-enhanced insulation offers substantial potential, its widespread adoption depends on cost-effectiveness and the availability of reliable production methods. Traditional methods will likely coexist with graphene-based solutions for some time.